CHAPERONE PROTEINS
Classical Chaperones
o Classical chaperones recognise hydrophobic segments of immature proteins on the outside of the protein that should be on the inside of the structure in the native state.
o They are regulated by adenine nucleotide binding and other co-factors.
o Chaperones work by providing a protected environment for proteins to fold correctly by minimising other interactions with the protein before it is fully folded.
Classical Chaperone Example
o An example of a classical chaperone is the HSP70 family- ATP hydrolysis dependent cycle that fold both misfolded and immature proteins. These have an N-terminal nucleotide binding domain (NBD) and C-terminal substrate binding domain (SBD).
o Substrate binding is regulated by a lid on the SBD that can open and close. ATP is bound to the lid when it is open giving a low affinity for the protein substrate. Hydrolysis of ATP closes the lid on the substrate giving the chaperone high affinity for the protein. This enclosed structure protects the protein from premature folding and forming other interactions before folding is complete.
o Cofactors such as BAP, SIL1 and nucleotide exchange factors can induce changes such as promoting the release of ADP causing the release of the protein once it has been folded, allowing for another ATP molecule to bind so the cycle can be repeated. BIP is the main regulator in the ER, binding to the majority of all proteins that pass through the ER at some point across the ER journey. Most proteins have a BIP binding site every 4 amino acids in their sequence.
Carbohydrate Chaperone Example
o An example of a carbohydrate chaperone is ER malectin. This chaperone binds to diglucosylated glycans in proteins, present in early stages of maturation.
The Unfolded Protein Response
When secretory proteins are misfolded, the ER needs to find a mode of coping with the added stress to maintain homeostasis of its environment. The unfolded protein response (UPR) is one of these coping mechanisms. Secretory proteins are constantly influxing into the ER, at a usual rate of about 0.1-1 million per minute per cell, posing a great challenge for correct protein folding and quality control. Many ER chaperone and accessory proteins aid in this. The availability of these chaperones depends on their concentration, substrate concentrations, duration of their associations, diffusion coefficients and time windows that they interact. When under stress, the ER becomes crowded, leading to an increased challenge for chaperones to find proteins before they misfold. The UPR therefore decreases the amount of proteins in the lumen, enhances the protein-folding capacity of the ER and promotes misfolded protein degradation [1]. Examples of chaperones include binding immunoglobulin protein (BiP/Grp78). Main functions of BiP involve translation slowing down and cell cycle arrest in the G1 phase within minutes of UPR activation to reduce further ER stress. It also maintains transmembrane protein sensors, binding to their luminal domain, rendering them inactive [2].
The ER possesses protein sensors detecting misfolded protein concentrations in the lumen. Upon accumulation of misfolded proteins, BiP molecules are recruited, dissociating from the transmembrane receptors, preventing them from forming aggregates, allowing the receptor to initiate downstream signalling of the UPR. BiP itself then binds to hydrophobic regions of misfolded proteins that are now exposed, activating a cascade of signals sent to both the nucleus and cytosol. Protein RNA-like ER kinase (PERK) is an example of these transmembrane protein sensors, dimerizing with BiP in resting cells, but oligomerizing with itself in stressed ones. It autophosphorylates its newly free luminal domain, activating its cytosolic domain to inhibit the translation of new proteins. Phosphorylation of α-subunit of the regulating initiator of mRNA translation machinery, eIF2α, does this [2].
The upregulation of UPR-initiating proteins leads to increased synthesis of proteins that chaperone misfolded proteins, making more BiP. These chaperones include inositol-requiring kinase 1 (IRE1) which in turn activates transcription factor XBP1, and ATF6. Misfolded proteins possess the tendency to accumulate and aggregate in the ER. If misfolded proteins pose continuous stress to the ER, proteins are guided through another stress coping pathway, the ERAD, where the chaperone EDEM guides the retro-translocation of misfolded proteins2. If these mechanisms are unsuccessful, a cellular apoptosis pathway is triggered so the translation machinery cannot make more misfiled proteins [3].
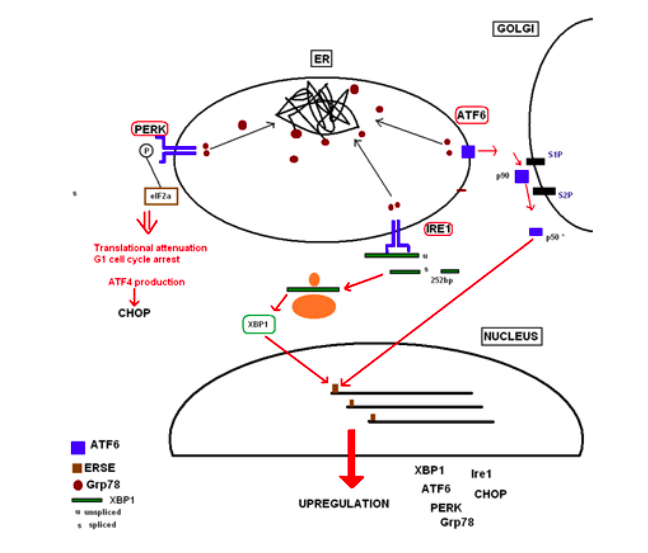